Berlin-Ichthyosaur State Park encompasses 1,540 acres. The elevation ranges from 6,840 feet to a high point of 7,880 feet. The hillsides in the park are covered with big sagebrush, while pinyon pine and Utah juniper dominate the upper elevations.
Common animal inhabitants include mule deer, black-tailed jackrabbits, cottontails, western bluebirds, pinyon jays, chukar partridge, whiptail lizards, western fence lizards, gophers and snakes.
Nestled at 7,000 feet on the western slope of central Nevada’s Shoshone mountain range, the park provides an array of stimulating recreational opportunities. The forested slopes provide shade, and breezes help to moderate the summer temperatures which seldom exceed 90˚F. Late spring and early fall can often be the nicest times of the year. Winter visits to the park are possible, but call for weather and road conditions before visiting.
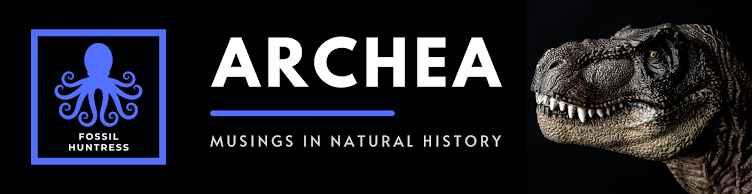
Monday 30 September 2019
Sunday 29 September 2019
PHYLLOCERAS OF JAPAN
Phylloceras consanguineum (Gemmellaro 1876) a fast-moving carnivorous ammonite from Late Jurassic (Middle Oxfordian) deposits near Sokoja, Madagasgar, off the southeast coast of Africa. (22.8° S, 44.4° E: 28.5° S, 18.2° E)
This classical Tethyan Mediterranean specimen is very well preserved, showing much of his delicate suturing in intricate detail. Phylloceras were primitive ammonites with involute, laterally flattened shells.
They were smooth, with very little ornamentation, which led researchers to think of them resembling plant leaves and gave rise to their name, which means "leaf-horn."
They can be found in three regions that I know of. In the Jurassic of Italy near western Sicily's Rosso Ammonitico Formation, Lower Kimmeridgian fossiliferous beds of Monte Inici East and Castello Inici (38.0° N, 12.9° E: 26.7° N, 15.4° E) and in the Armine area, southeastern Toyama Prefecture, northern central Japan, roughly (36.5° N, 137.5° E: 43.6° N, 140.6° E).
This classical Tethyan Mediterranean specimen is very well preserved, showing much of his delicate suturing in intricate detail. Phylloceras were primitive ammonites with involute, laterally flattened shells.
They were smooth, with very little ornamentation, which led researchers to think of them resembling plant leaves and gave rise to their name, which means "leaf-horn."
They can be found in three regions that I know of. In the Jurassic of Italy near western Sicily's Rosso Ammonitico Formation, Lower Kimmeridgian fossiliferous beds of Monte Inici East and Castello Inici (38.0° N, 12.9° E: 26.7° N, 15.4° E) and in the Armine area, southeastern Toyama Prefecture, northern central Japan, roughly (36.5° N, 137.5° E: 43.6° N, 140.6° E).
Saturday 28 September 2019
OUR CARIBOO MOUNTAINS
We soak up the breathtaking views after a long morning's paddle. The east and south sides of our route are bound by the imposing white peaks of the Cariboo Mountains, the northern boundary of the Interior wet belt, rising up across the Rocky Mountain Trench, and the Isaac Formation, the oldest of seven formations that make up the Cariboo Group.
Some 270 million-plus years ago, had one wanted to buy waterfront property in what is now British Columbia, you’d be looking somewhere between Prince George and the Alberta border. The rest of the province had yet to arrive but would be made up of over twenty major terranes from around the Pacific. The rock that would eventually become the Cariboo Mountains and form the lakes and valleys of Bowron was far out in the Pacific Ocean, down near the equator.
With tectonic shifting, these rocks drifted north-eastward, riding their continental plate, until they collided with and joined the Cordillera in what is now British Columbia. Continued pressure and volcanic activity helped create the tremendous slopes of the Cariboo Range we see today with repeated bouts of glaciation during the Pleistocene carving their final shape. Warm and dry with bellies filled full of soup and crisps, we head back out to explore more of nature's bounty.
Some 270 million-plus years ago, had one wanted to buy waterfront property in what is now British Columbia, you’d be looking somewhere between Prince George and the Alberta border. The rest of the province had yet to arrive but would be made up of over twenty major terranes from around the Pacific. The rock that would eventually become the Cariboo Mountains and form the lakes and valleys of Bowron was far out in the Pacific Ocean, down near the equator.
With tectonic shifting, these rocks drifted north-eastward, riding their continental plate, until they collided with and joined the Cordillera in what is now British Columbia. Continued pressure and volcanic activity helped create the tremendous slopes of the Cariboo Range we see today with repeated bouts of glaciation during the Pleistocene carving their final shape. Warm and dry with bellies filled full of soup and crisps, we head back out to explore more of nature's bounty.
Friday 27 September 2019
HOLCOPHYLLOCERAS OF MADAGASCAR
There is tremendously robust suturing on this lovely ammonite, Holcophylloceras mediterraneum, (Neumayr 1871) from Late Jurassic (Oxfordian) deposits near Sokoja, Madagasgar. Madagascar is a treasure trove of outstanding fossil species and this ammonite is no exception.
The shells had many chambers divided by walls called septa. The chambers were connected by a tube called a siphuncle which allowed for the control of buoyancy with the hollow inner chambers of the shell acting as air tanks to help them float.
We can see the edges of this specimen's shell where it would have continued out to the last chamber, the body chamber, where the ammonite lived. Picture a squid or octopus, now add a shell and a ton of water.
The shells had many chambers divided by walls called septa. The chambers were connected by a tube called a siphuncle which allowed for the control of buoyancy with the hollow inner chambers of the shell acting as air tanks to help them float.
We can see the edges of this specimen's shell where it would have continued out to the last chamber, the body chamber, where the ammonite lived. Picture a squid or octopus, now add a shell and a ton of water.
Thursday 26 September 2019
EOCENE INSECTS OF PRINCETON
March Flies are hardy, medium-sized flies in the Order Diptera, with a body length ranging from 4.0 to 10.0 mm. This species is one of the most satisfying fossils to collect in the Eocene deposits of Princeton, British Columbia.
The body is black, brown, or rusty, and thickset, with thick legs. The antennae are moniliform. The front tibiae bear large strong spurs or a circlet of spines. The tarsi are five-segmented and bear tarsal claws, pulvilli, and a well-developed empodium. As it is with many species, these guys included, the teens of this species are troublesome but the adults turn out alright. As larvae, Bibionidae is an agricultural pest, devouring all those tasty young seedlings you've just planted.
Then, as they mature their tastes turn to the nectar of flowers from fruit trees and la voila, they become your best friends again. With their physical and behavioural transformation complete, Bibionidae becomes a welcome garden visitor, pulling their weight in the ecosystems they live in by being important pollinators.
The body is black, brown, or rusty, and thickset, with thick legs. The antennae are moniliform. The front tibiae bear large strong spurs or a circlet of spines. The tarsi are five-segmented and bear tarsal claws, pulvilli, and a well-developed empodium. As it is with many species, these guys included, the teens of this species are troublesome but the adults turn out alright. As larvae, Bibionidae is an agricultural pest, devouring all those tasty young seedlings you've just planted.
Then, as they mature their tastes turn to the nectar of flowers from fruit trees and la voila, they become your best friends again. With their physical and behavioural transformation complete, Bibionidae becomes a welcome garden visitor, pulling their weight in the ecosystems they live in by being important pollinators.
Wednesday 25 September 2019
GIANT GROUND SLOTH
In 1788, this magnificent specimen of a Megatherium sloth was sent to the Royal Cabinet of Natural History from the Viceroyalty of Rio de la Plata.
The megaterios were large terrestrial sloths belonging to the group, Xenarthra. These herbivores inhabited large areas of land on the American continent. Their powerful skeleton enabled them to stand on their hind legs to reach leaves high in the trees, a huge advantage given the calories needed to be consumed each day to maintain their large size.
Avocados were one of the food preferences of our dear Giant ground sloths. They ate then pooped them out, spreading the pits far and wide. The next time you enjoy avocado toast, thank this large beastie. One of his ancestors may have had a hand (or butt) in your meal.
In 1788, Bru assembled the skeleton as you see it here. It is exhibited at the Museo Nacional De Ciencias Naturales in Madrid, Spain, in its original configuration for historic value. If you look closely, you'll see it is not anatomically correct. But all good paleontology is teamwork. Based upon the drawings of Juan Bautista Bru, George Cuvier used this specimen to describe the species for the very first time.
The megaterios were large terrestrial sloths belonging to the group, Xenarthra. These herbivores inhabited large areas of land on the American continent. Their powerful skeleton enabled them to stand on their hind legs to reach leaves high in the trees, a huge advantage given the calories needed to be consumed each day to maintain their large size.
Avocados were one of the food preferences of our dear Giant ground sloths. They ate then pooped them out, spreading the pits far and wide. The next time you enjoy avocado toast, thank this large beastie. One of his ancestors may have had a hand (or butt) in your meal.
In 1788, Bru assembled the skeleton as you see it here. It is exhibited at the Museo Nacional De Ciencias Naturales in Madrid, Spain, in its original configuration for historic value. If you look closely, you'll see it is not anatomically correct. But all good paleontology is teamwork. Based upon the drawings of Juan Bautista Bru, George Cuvier used this specimen to describe the species for the very first time.
Tuesday 24 September 2019
MIDDLE TRIASSIC AMMONOID & BELEMNITE
A beautiful plate with a lovely example of the Middle Triassic ammonoid and belemnite from Fossil Hill in the Humboldt Mountains of Nevada. This locality is perhaps the most famous and important locality for the Middle Triassic (Anisian/Ladinian) of North America.
This was the site of the 1905 Expedition of the University of California’s Department of Geology in Berkeley funded by the beautiful and bold, Annie Alexander, the women to whom the UCMP owes both its collection and existence. J. P. Smith joined the expedition, though he was interested in the invertebrate fauna, not the mighty marine reptiles that helped get the project funded.
J.P. Smith published on the marine fauna in the early 1900s. They formed the basis for his monograph on North American Middle Triassic marine invertebrate fauna published in 1914.
N. J. Siberling from the US Geological Survey published on these outcrops in 1962. His work included nearly a dozen successive ammonite faunas, many of which were variants on previously described species. Evidently, his collections consisted mainly of weathered material and were made without stratigraphic control because he believed that most, if not all, of these species, were coexistent. The fossiliferous beds found here, as well as localities in north-western Nevada, were designated the 'Daonella dubia' zone. Dubious would be closer to the truth. Smith joined the 1905 Expedition of the University of California’s Department of Geology in Berkeley funded by the beautiful and bold, Annie Alexander, the women to whom the UCMP owes both its collection and existence.
This was the site of the 1905 Expedition of the University of California’s Department of Geology in Berkeley funded by the beautiful and bold, Annie Alexander, the women to whom the UCMP owes both its collection and existence. J. P. Smith joined the expedition, though he was interested in the invertebrate fauna, not the mighty marine reptiles that helped get the project funded.
J.P. Smith published on the marine fauna in the early 1900s. They formed the basis for his monograph on North American Middle Triassic marine invertebrate fauna published in 1914.
N. J. Siberling from the US Geological Survey published on these outcrops in 1962. His work included nearly a dozen successive ammonite faunas, many of which were variants on previously described species. Evidently, his collections consisted mainly of weathered material and were made without stratigraphic control because he believed that most, if not all, of these species, were coexistent. The fossiliferous beds found here, as well as localities in north-western Nevada, were designated the 'Daonella dubia' zone. Dubious would be closer to the truth. Smith joined the 1905 Expedition of the University of California’s Department of Geology in Berkeley funded by the beautiful and bold, Annie Alexander, the women to whom the UCMP owes both its collection and existence.
Monday 23 September 2019
AN ANCIENT MARINE LINEAGE
Life on Earth began in the oceans more than 3.5 billion years ago. That means that all of us, all of our homo sapien sapien brethren and every living thing on the planet is a descendant of a marine organism.
Knowing that we share an ancient history with every living species is rather humbling. While we diverged early on from cats, dogs, frogs and birds, we share a history and proud lineage with all the vertebrates alive today.
I was up hunting for trilobites near Cranbrook and came across a graptolite. It looked like a wee pen mark on a bit of rock. It too is one of our relatives and one of the earliest. So how did vertebrates go from worm-like marine animals like Metaspriggina, to the modern diversity of forms?
Knowing that we share an ancient history with every living species is rather humbling. While we diverged early on from cats, dogs, frogs and birds, we share a history and proud lineage with all the vertebrates alive today.
I was up hunting for trilobites near Cranbrook and came across a graptolite. It looked like a wee pen mark on a bit of rock. It too is one of our relatives and one of the earliest. So how did vertebrates go from worm-like marine animals like Metaspriggina, to the modern diversity of forms?
Friday 20 September 2019
CRETACEOUS NAUTILUS OF MEXICO
A picture-perfect Campanian nautilus, Eutrophoceras irritilansis,
who lived during the Upper Cretaceous (late Campanian) near the town of Porvenir de Jalpa (about 64 km / 40 miles west of Saltillo) in what is now southern Coahuila (formerly Coahuila de Zaragoza), northern Mexico. Collection of Jose Ventura.
who lived during the Upper Cretaceous (late Campanian) near the town of Porvenir de Jalpa (about 64 km / 40 miles west of Saltillo) in what is now southern Coahuila (formerly Coahuila de Zaragoza), northern Mexico. Collection of Jose Ventura.
Thursday 19 September 2019
FERGUSON HILL, NEVADA
Ferguson Hill contains the most complete macrofossil record spanning the Triassic-Jurassic boundary in North America. The ammonoids from the uppermost Triassic can be traced to the boundary and the earliest ammonites (Psiloceratids) can be seen right at the base of the Jurassic (Hettangian).
It was in contention for the Global Stratotype Section and Point (GSSP) defining the base of the Jurassic System, Lower Jurassic Series and Hettangian Stage. However, in 2008, the Kuhjoch (Karwendel Mountains, Northern Calcareous Alps, Tyrol, Austria) was chosen over Ferguson Hill mainly because the beds containing the oldest Psiloceras (P. spelae spelae) were better preserved and contained Hettangian microfossils.
It was in contention for the Global Stratotype Section and Point (GSSP) defining the base of the Jurassic System, Lower Jurassic Series and Hettangian Stage. However, in 2008, the Kuhjoch (Karwendel Mountains, Northern Calcareous Alps, Tyrol, Austria) was chosen over Ferguson Hill mainly because the beds containing the oldest Psiloceras (P. spelae spelae) were better preserved and contained Hettangian microfossils.
Wednesday 18 September 2019
ROCKY MOUNTAIN COUGAR
Cougars are meat-eating mammals (primarily dining on deer) who boast being the most widely distributed land mammal in the Western Hemisphere. They are impressive athletes, able to leap 18 feet or more straight upward from a sitting position.
They lead solitary lives and are excellent at avoiding humans for the most part. Cougars have a massive range that runs from the mountainous Canadian Rockies in northwestern Canada all the way down to Patagonia in South America. These cats make their dens in mountain graggs, along rocky ledges, in dense woodland areas and under uprooted trees and debris.
They lead solitary lives and are excellent at avoiding humans for the most part. Cougars have a massive range that runs from the mountainous Canadian Rockies in northwestern Canada all the way down to Patagonia in South America. These cats make their dens in mountain graggs, along rocky ledges, in dense woodland areas and under uprooted trees and debris.
Monday 16 September 2019
FOSSIL RELIC: AMIIFORMES
This well-preserved fossil fish skull is from Calamopleurus (Agassiz, 1841), an extinct genus of bony fishes related to the heavily armoured ray-finned gars.
They are fossil relics, the sole surviving species of the order Amiiformes. Although bowfins are highly evolved, they are often referred to as primitive fishes and living fossils as they retain many of the morphologic characteristics of their ancestors.
This specimen was found in Lower Cretaceous outcrops of the Santana Formation in the Araripe Basin UNESCO Global Geopark of northeastern Brazil. Collection of David Murphy
They are fossil relics, the sole surviving species of the order Amiiformes. Although bowfins are highly evolved, they are often referred to as primitive fishes and living fossils as they retain many of the morphologic characteristics of their ancestors.
This specimen was found in Lower Cretaceous outcrops of the Santana Formation in the Araripe Basin UNESCO Global Geopark of northeastern Brazil. Collection of David Murphy
Sunday 15 September 2019
NOTOCHORDS: CHORDATES TO CALAMARI
You and I are vertebrates, we have backbones. Having a backbone or spinal column is what sets apart you, me and almost 70,000 species on this big blue planet.
So which lucky ducks evolved one? Well, ducks for one. Warm-blooded birds and mammals cheerfully claim those bragging rights. They're joined by our cold-blooded, ectothermic friends, the fish, amphibians and reptiles. All these diverse lovelies share this characteristic.
And whether they now live at sea or on land, all of these lineages evolved from a marine organism somewhere down the line, then went on to develop a notochord and spinal column. Notochords are flexible rods that run down the length of chordates and vertebrates. They are handy adaptations for muscle attachment, helping with signalling and coordinating the development of the embryonic stage. The cells from the notochord play a key role in the development of the central nervous system and the formation of motor neurons and sensory cells. Alas, we often take our evolution for granted.
Let's take a moment to appreciate just how marvellous this evolutionary gift is and what it allows us to do. Your backbone gives your body structure, holds up that heavy skull of yours and connects your tasty brain to your body and organs. Eating, walking, fishing, hunting, your morning yoga class, are all made possible because of this adaptation. Pick pretty near anything you love to do and it is only possible because of your blessed spine.
And it sets us apart from our invertebrate friends.
While seventy thousand may seem like a large number, it represents less than three to five per cent of all described animal species. The rest is made up of the whopping 97%'ers, our dear invertebrates who include the arthropods (insects, arachnids, crustaceans, and myriapods), molluscs (our dear chitons, snails, bivalves, squid, and octopus), annelids (the often misunderstood earthworms and leeches), and cnidarians (our beautiful hydras, jellyfish, sea anemones, and corals). You'll notice that many of our invertebrate friends occur as tasty snacks. Having a backbone provides a supreme advantage to your placement in the food chain. Not always, as you may include fish and game on your menu. But generally, having a backbone means you're more likely to be holding the menu versus being listed as an appetizer. So, enjoy your Sunday 'downward dog' and thank your backbone for the magical gift it is.
So which lucky ducks evolved one? Well, ducks for one. Warm-blooded birds and mammals cheerfully claim those bragging rights. They're joined by our cold-blooded, ectothermic friends, the fish, amphibians and reptiles. All these diverse lovelies share this characteristic.
And whether they now live at sea or on land, all of these lineages evolved from a marine organism somewhere down the line, then went on to develop a notochord and spinal column. Notochords are flexible rods that run down the length of chordates and vertebrates. They are handy adaptations for muscle attachment, helping with signalling and coordinating the development of the embryonic stage. The cells from the notochord play a key role in the development of the central nervous system and the formation of motor neurons and sensory cells. Alas, we often take our evolution for granted.
Let's take a moment to appreciate just how marvellous this evolutionary gift is and what it allows us to do. Your backbone gives your body structure, holds up that heavy skull of yours and connects your tasty brain to your body and organs. Eating, walking, fishing, hunting, your morning yoga class, are all made possible because of this adaptation. Pick pretty near anything you love to do and it is only possible because of your blessed spine.
And it sets us apart from our invertebrate friends.
While seventy thousand may seem like a large number, it represents less than three to five per cent of all described animal species. The rest is made up of the whopping 97%'ers, our dear invertebrates who include the arthropods (insects, arachnids, crustaceans, and myriapods), molluscs (our dear chitons, snails, bivalves, squid, and octopus), annelids (the often misunderstood earthworms and leeches), and cnidarians (our beautiful hydras, jellyfish, sea anemones, and corals). You'll notice that many of our invertebrate friends occur as tasty snacks. Having a backbone provides a supreme advantage to your placement in the food chain. Not always, as you may include fish and game on your menu. But generally, having a backbone means you're more likely to be holding the menu versus being listed as an appetizer. So, enjoy your Sunday 'downward dog' and thank your backbone for the magical gift it is.
Saturday 14 September 2019
HEXAGONAL COLUMNS: COOLING BASALT
The Giant's Causeway is a spectacular expanse of interlocking hexagonal basalt columns formed from volcanic eruptions during the Paleocene some 50-60 million years ago.
These columns tell a story of the cooling and freezing of the lava flows that formed them. As lava at the surface cools and freezes, it also shrinks as its molecules rearrange themselves into a solid structure. This happens much more quickly at the surface where the lava comes in contact with moist, cool air. As the basalt cools and shrinks, pressure increases in intensity and cracks begin to form. A way to dissipate this huge stress is to crack at an angle of 120 degrees, the angle that gives us a hexagon.
We see this beautifully illustrated at the Giant's Causeway in Ireland. Here, highly fluid molten basalt intruded through chalk beds which later cooled, contracted and cracked into hexagonal columns, creating a surreal visual against a dark and stormy Irish Sea.
These columns tell a story of the cooling and freezing of the lava flows that formed them. As lava at the surface cools and freezes, it also shrinks as its molecules rearrange themselves into a solid structure. This happens much more quickly at the surface where the lava comes in contact with moist, cool air. As the basalt cools and shrinks, pressure increases in intensity and cracks begin to form. A way to dissipate this huge stress is to crack at an angle of 120 degrees, the angle that gives us a hexagon.
We see this beautifully illustrated at the Giant's Causeway in Ireland. Here, highly fluid molten basalt intruded through chalk beds which later cooled, contracted and cracked into hexagonal columns, creating a surreal visual against a dark and stormy Irish Sea.
Friday 13 September 2019
DORIPPE SINICA OF JAPAN
A beautiful example of the decapod, Dorippe sinica, from Holocene deposits near Shizuoka, Japan. This regal fellow has a strongly sculptured carapace. He looks like he would have been quite the bruiser moving about on the seafloor looking for tasty snacks. He likely enjoyed just about any form of meat, potentially dining on fish, worms, eggs, squid, starfish or even a few of his slow-moving cousins.
The carapace is deeply grooved with conspicuous wart-like tubercles; anterolateral margin, between the base of the exorbital tooth and cervical groove, smooth, without tubercles or denticles.
The teeth on the lower orbital margin in the cluster. Carpus of cheliped distinctly granulated on the upper surface and with a conspicuous row of granules along the anterior margin. Though missing here, the merus of second and third pereiopods are almost cylindrical. (Türkay 1995). This specimen was collected and is the collection of the deeply awesome Takashi Ito of Japan
The carapace is deeply grooved with conspicuous wart-like tubercles; anterolateral margin, between the base of the exorbital tooth and cervical groove, smooth, without tubercles or denticles.
The teeth on the lower orbital margin in the cluster. Carpus of cheliped distinctly granulated on the upper surface and with a conspicuous row of granules along the anterior margin. Though missing here, the merus of second and third pereiopods are almost cylindrical. (Türkay 1995). This specimen was collected and is the collection of the deeply awesome Takashi Ito of Japan
ARANDASPIDA: AGENTS OF SHIELD
The oldest and most primitive pteraspidomorphs were the Astraspida and the Arandaspida. You'll notice that all three of these taxon names contain 'aspid', which means shield.
These early fishes and many of the Pteraspidomorphi possessed large plates of dermal bone at the anterior end of their bodies. This dermal armour was very common in early vertebrates, but it was lost in their descendants. Arandaspida is represented by two well-known genera: Sacabampaspis, from South America and Arandaspis from Australia. Arandaspis have large, simple, dorsal and ventral head shields. Their bodies were fusiform, which means they were shaped sort of like a spindle, fat in the middle and tapering at both ends. Picture a sausage that is a bit wider near the centre with a crisp outer shell. Image: Tamura (http://spinops.blogspot.com) - Own work, CC BY-SA 3.0, https://commons.wikimedia.org/w/index.php?curid=19460450
These early fishes and many of the Pteraspidomorphi possessed large plates of dermal bone at the anterior end of their bodies. This dermal armour was very common in early vertebrates, but it was lost in their descendants. Arandaspida is represented by two well-known genera: Sacabampaspis, from South America and Arandaspis from Australia. Arandaspis have large, simple, dorsal and ventral head shields. Their bodies were fusiform, which means they were shaped sort of like a spindle, fat in the middle and tapering at both ends. Picture a sausage that is a bit wider near the centre with a crisp outer shell. Image: Tamura (http://spinops.blogspot.com) - Own work, CC BY-SA 3.0, https://commons.wikimedia.org/w/index.php?curid=19460450
Thursday 12 September 2019
TYAUGHTON FOSSIL EXPOSURES
Our collecting area near the Tyaughton Fossil Exposures, Taseko Lakes, British Columbia, Canada. Tyaughton is kind of like El Dorado. Instead of immeasurable riches in gold, this region of the Chilcotin Mountains holds the treasures of time — bountiful Triassic-Jurassic fossils
TIKTAALIK OF ELLESMERE ISLAND
Tiktaalik was a fish with some advantageous tetrapod-like features that proved to be useful to an adaptation to land. Their head was detached from their shoulder girdle meaning they could lift their heads to take a look around. This was a new adaptation for our marine friends. The bones in the front limb were strong enough and adapted to support their bodies.
Tuesday 10 September 2019
PAKICETUS: UNLIKELY WHALES
The unlikely creature bearing the title of "the first whale," is a fellow named Pakicetus. He is definitely not how we picture whales living today. Pakicetus is an extinct genus of cetaceans that lived about 50 million years ago.
They were mammals and looked like large rodents. They were also quite small by whale standards, reaching about four-feet in length. They ate meat, sometimes fish and are the ancestors of whales, porpoises and dolphins.
The only real clue of their connection to our aquatic friends is the shape of their skulls. Pakicetus had a long skull and an ear bone that is unique to whales. Oddly, they also had ankle bones that share characteristics with some of our even-toed mammals. They lived along the shores of a large shallow sea known as the Tethys. Although rare, there are several examples of mammals heading back to a life at sea. Photo: Kevin Guertin from Ottawa, Ontario, Canada - DSCF1201, CC BY-SA 2.0, https://commons.wikimedia.org/w/index.php?curid=36657302
They were mammals and looked like large rodents. They were also quite small by whale standards, reaching about four-feet in length. They ate meat, sometimes fish and are the ancestors of whales, porpoises and dolphins.
The only real clue of their connection to our aquatic friends is the shape of their skulls. Pakicetus had a long skull and an ear bone that is unique to whales. Oddly, they also had ankle bones that share characteristics with some of our even-toed mammals. They lived along the shores of a large shallow sea known as the Tethys. Although rare, there are several examples of mammals heading back to a life at sea. Photo: Kevin Guertin from Ottawa, Ontario, Canada - DSCF1201, CC BY-SA 2.0, https://commons.wikimedia.org/w/index.php?curid=36657302
Monday 9 September 2019
DENMAN ISLAND CONCRETION
![]() |
Northumberland Fm, Upper Cretaceous Nanaimo Group |
Thee Upper Cretaceous Nanaimo Group of southwest British Columbia is a >4 km-thick succession consisting mostly of deep marine siliciclastics deposited directly on the Insular Superterrane. As such, this succession has been the focus of several paleomagnetic, isotope geochemistry, paleontology, and sedimentology studies in attempts to elucidate the tectonic history and paleolatitude of the Insular Superterrane and associated entities during the critical time of Nanaimo Group deposition, 90 to 65 million years ago. The upper two-thirds of the succession is continuously and well exposed on Denman and Hornby islands and represents the best example of this part of the succession in the northern half of what we consider the single Nanaimo Basin. A concretion found on the beach at Denman, eroding out of the grey shales of the Upper Cretaceous Nanaimo Group
Sunday 8 September 2019
NOOTKA ISLAND
![]() |
Nootka Sound, Photo: Dan Bowen |
The area is known for its exceptional natural beauty and bounty of beautifully preserved decapod fossil specimens. The formation is named for the Hesquiaht people of the Nuu-chah-nulth, of Nootka Sound. The VIPS has led many research expeditions to remote sites on our West Coast. Their efforts have been rewarded with many new species being identified and excellent cooperation with paleontological researchers from around the globe.
Friday 6 September 2019
BIODIVERSITY AT SEA AND ON LAND
Most of the Earth's surface is an ocean. When I think of the Earth, it is our oceans that I picture. Life began there. We began there. Most of the major animal groups can trace their lineage back to the seas and the Cambrian explosion, an orgy of breathtaking species diversification.
Since that time, a shocking half a billion years ago, our seas have played host to an astonishing array of species. If I'd visited our Earth back in the Cambrian, I would have bet good money that our watery planet's future was in the seas not on the land. But that 's not the case. Quite surprisingly, it is our humble rock and soil who now boast more species. Five times that of those living in the oceans. I know, shocking but true. Our oceans certainly had the running start on both numbers and diversity of species. But it is our fungi, our flowering plants, mindblowing variety of insects, trees, bees and fleas that make up the bulk of Earth's species these days.
It is something I'm interested in learning more about as it does not make good sense to me. 80 percent of Earth's species live on land today. About 15 percent call our oceans home and another 5% or so live in freshwater. Why more species live on land than in the ocean has puzzled others as well. Robert May, a zoologist at the University of Oxford, mulls this very question in an article from 1994 titled, “Biological Diversity: Differences between Land and Sea.” He continued with his research and published "The future of biological diversity in a crowded world," in Current Science, Vol. 82, No. 11 (10 June 2002), pp. 1325-1331.
Here he questions how well we know the plants, animals and micro-organisms with which we share this beautiful planet. His focus in the paper was to question how many species are there and how fast are some going extinct? You'll be interested to know that his best guess in 2002 was somewhere between 1.7 to 1.8 million. That's a considerable increase from Carl Linneaus' work back in 1758, the Swedish botanist, zoologist, and physician took a stab at the same question and came up with an estimate of about 9,000 species. While his numbers were off by a long margin, he did give us the binomial nomenclature system we use for naming organisms, so he still gets a hall pass.
May is a boy about town. His work is referenced everywhere. You may enjoy an article by the Atlantic from 2017 that delves into the topic for the lay audience with an eye to popularized reading. May, R. (2002). The future of biological diversity in a crowded world. Current Science, 82(11), 1325-1331. Retrieved from http://www.jstor.org/stable/24105996 / The Atlantic article: https://www.theatlantic.com/science/archive/2017/07/why-are-there-so-many-more-species-on-land-than-in-the-sea/533247/
Since that time, a shocking half a billion years ago, our seas have played host to an astonishing array of species. If I'd visited our Earth back in the Cambrian, I would have bet good money that our watery planet's future was in the seas not on the land. But that 's not the case. Quite surprisingly, it is our humble rock and soil who now boast more species. Five times that of those living in the oceans. I know, shocking but true. Our oceans certainly had the running start on both numbers and diversity of species. But it is our fungi, our flowering plants, mindblowing variety of insects, trees, bees and fleas that make up the bulk of Earth's species these days.
It is something I'm interested in learning more about as it does not make good sense to me. 80 percent of Earth's species live on land today. About 15 percent call our oceans home and another 5% or so live in freshwater. Why more species live on land than in the ocean has puzzled others as well. Robert May, a zoologist at the University of Oxford, mulls this very question in an article from 1994 titled, “Biological Diversity: Differences between Land and Sea.” He continued with his research and published "The future of biological diversity in a crowded world," in Current Science, Vol. 82, No. 11 (10 June 2002), pp. 1325-1331.
Here he questions how well we know the plants, animals and micro-organisms with which we share this beautiful planet. His focus in the paper was to question how many species are there and how fast are some going extinct? You'll be interested to know that his best guess in 2002 was somewhere between 1.7 to 1.8 million. That's a considerable increase from Carl Linneaus' work back in 1758, the Swedish botanist, zoologist, and physician took a stab at the same question and came up with an estimate of about 9,000 species. While his numbers were off by a long margin, he did give us the binomial nomenclature system we use for naming organisms, so he still gets a hall pass.
May is a boy about town. His work is referenced everywhere. You may enjoy an article by the Atlantic from 2017 that delves into the topic for the lay audience with an eye to popularized reading. May, R. (2002). The future of biological diversity in a crowded world. Current Science, 82(11), 1325-1331. Retrieved from http://www.jstor.org/stable/24105996 / The Atlantic article: https://www.theatlantic.com/science/archive/2017/07/why-are-there-so-many-more-species-on-land-than-in-the-sea/533247/
Wednesday 4 September 2019
FAVRET CANYON
Favret Canyon is considered one of the most important locations for the Middle Triassic (Anisian-Ladinian). It is a beautiful, yet desolate country. The roads are rough and the exposures are weathered out. The mountains bring a moodiness to the landscape and impact the weather.
We had stormy clouds, rain, sun, and afternoons of wind while walking through time and collecting marine fauna.
We had stormy clouds, rain, sun, and afternoons of wind while walking through time and collecting marine fauna.
Tuesday 3 September 2019
PRIMITIVE FISH OF THE CHENGJIANG LAGERSTÄTTE
Three genera of Lower Cambrian fish are known from the 530 million-year-old Chengjiang Lagerstätte in Yuxi, Yunnan Province, southern China. The locality is just north of Fuxian Lake and about a half-hour drive south from the city of Kunming.
A friend of mine, Eldon Grupp from the USA, found an 18 mm specimen on a mortality slab of Haikouella from Chengjiang. Apparently, no one had noticed it before shipping. Not surprising as Zhongjianichthys are easy to overlook. I've asked him if I can get a photo of that mortality plate to share with you. It's quite stunning. Haikouella, of course, are not vertebrates, but advanced craniate chordates. The specimen in question, however, was a vertebrate. Eldon has assigned this specimen to genus Zhongjianichthys based on its eel-like characteristics and its large eyes located behind the anterior or rostral lobe instead of within it. Even so, family affiliation is uncertain.
- Haikouichthys ercaicunensis
- Myllokunmingia fengjiaoa
- Zhongjianichthys rostratus
A friend of mine, Eldon Grupp from the USA, found an 18 mm specimen on a mortality slab of Haikouella from Chengjiang. Apparently, no one had noticed it before shipping. Not surprising as Zhongjianichthys are easy to overlook. I've asked him if I can get a photo of that mortality plate to share with you. It's quite stunning. Haikouella, of course, are not vertebrates, but advanced craniate chordates. The specimen in question, however, was a vertebrate. Eldon has assigned this specimen to genus Zhongjianichthys based on its eel-like characteristics and its large eyes located behind the anterior or rostral lobe instead of within it. Even so, family affiliation is uncertain.
Monday 2 September 2019
SIRENIA: MANATEES AND DUGONGS
I'd always grouped the dugongs and manatees together. There are slight differences between these two groups. Both groups belong to the order Sirenia. They shared a cousin in the Steller's sea cow, Hydrodamalis gigas, but that piece of their lineage was hunted to extinction by our species in the 18th century. Dugongs have tail flukes with pointed tips and manatees have paddle-shaped tails, similar to a Canadian Beaver.
Both of these lovelies from the order Sirenia went from terrestrial to marine, taking to the water in search of more prosperous pastures, as it were.
They are the extant and extinct forms of the oddball manatees and dugongs. They inhabit rivers and shallow coastal waters, making the best use of their fusiform bodies that lack dorsal fins and hind limbs. I've been thinking about them in the context of some of the primitive armoured fish we find in the Chengjiang biota of China, specifically those primitive species that were also fusiform.
We find dugongs today in waters near northern Australia and parts of the Indian and Pacific Oceans. They favour locations where seagrass, their food of choice, grows plentiful and they eat it roots and all. While seagrass low in fibre, high in nitrogen and easily digestible is preferred, dugongs will also dine on lower grade seagrass, algae and invertebrates should the opportunity arise. They've been known to eat jellyfish, sea squirts and shellfish over the course of their long lives. Some of the oldest dugongs have been known to live 70+ years, which is another statistic I find surprising. They are large, passive, have poor eyesight and look pretty tasty floating in the water; a defenceless floating buffet. Their population is in decline but yet they live on.
Both of these lovelies from the order Sirenia went from terrestrial to marine, taking to the water in search of more prosperous pastures, as it were.
They are the extant and extinct forms of the oddball manatees and dugongs. They inhabit rivers and shallow coastal waters, making the best use of their fusiform bodies that lack dorsal fins and hind limbs. I've been thinking about them in the context of some of the primitive armoured fish we find in the Chengjiang biota of China, specifically those primitive species that were also fusiform.
We find dugongs today in waters near northern Australia and parts of the Indian and Pacific Oceans. They favour locations where seagrass, their food of choice, grows plentiful and they eat it roots and all. While seagrass low in fibre, high in nitrogen and easily digestible is preferred, dugongs will also dine on lower grade seagrass, algae and invertebrates should the opportunity arise. They've been known to eat jellyfish, sea squirts and shellfish over the course of their long lives. Some of the oldest dugongs have been known to live 70+ years, which is another statistic I find surprising. They are large, passive, have poor eyesight and look pretty tasty floating in the water; a defenceless floating buffet. Their population is in decline but yet they live on.
Sunday 1 September 2019
THE LURE OF THE SEA
Many land animals have returned to the sea throughout evolutionary history. We have beautifully documented cases from amphibians, reptiles, birds and mammals from over 30 different lineages over the past 250 million years.
Our dear penguins, seals, sea lions, walruses, whales, crocodiles and sea turtles were once entirely terrestrial. Some dipped a toe or two into freshwater ponds, but make no mistake, they were terrestrial. Each of these animals had ancestors that tried out the sea and decided to stay. They evolved and employed a variety of adaptations to meet their new saltwater challenges. Some adapted legs as fins, others became more streamlined, and still, others developed specialized organs to extract dissolved oxygen from the water through their skin or gills. The permutations are endless.
Returning to the sea comes with a whole host of benefits but some serious challenges as well. Life at sea is very different from life on land. Water is denser than air, impacting how an animal moves, sees and hears. More importantly, it impacts an air-breathing animal's movement on a pretty frequent basis. If you need air and haven't evolved gills, you need to surface frequently. Keeping your body temperature at a homeostatic level is also a challenge as water conducts heat much better than air. Even with all of these challenges, the lure of additional food sources and freedom of movement kept those who tried the sea in the sea and they evolved accordingly.
This is an interesting article from Alicia Ault writing for the Smithsonian who interviewed Nick Pysenson and Neil Kelley about some of their research that touches on this area. They published a paper on it in the journal Science back in 2015.
Here's the link: https://science.sciencemag.org/content/348/6232/aaa3716
And Ault's work is definitely worth a read: https://www.smithsonianmag.com/smithsonian-institution/take-deep-dive-reasons-land-animals-moved-seas-180955007/
Our dear penguins, seals, sea lions, walruses, whales, crocodiles and sea turtles were once entirely terrestrial. Some dipped a toe or two into freshwater ponds, but make no mistake, they were terrestrial. Each of these animals had ancestors that tried out the sea and decided to stay. They evolved and employed a variety of adaptations to meet their new saltwater challenges. Some adapted legs as fins, others became more streamlined, and still, others developed specialized organs to extract dissolved oxygen from the water through their skin or gills. The permutations are endless.
Returning to the sea comes with a whole host of benefits but some serious challenges as well. Life at sea is very different from life on land. Water is denser than air, impacting how an animal moves, sees and hears. More importantly, it impacts an air-breathing animal's movement on a pretty frequent basis. If you need air and haven't evolved gills, you need to surface frequently. Keeping your body temperature at a homeostatic level is also a challenge as water conducts heat much better than air. Even with all of these challenges, the lure of additional food sources and freedom of movement kept those who tried the sea in the sea and they evolved accordingly.
This is an interesting article from Alicia Ault writing for the Smithsonian who interviewed Nick Pysenson and Neil Kelley about some of their research that touches on this area. They published a paper on it in the journal Science back in 2015.
Here's the link: https://science.sciencemag.org/content/348/6232/aaa3716
And Ault's work is definitely worth a read: https://www.smithsonianmag.com/smithsonian-institution/take-deep-dive-reasons-land-animals-moved-seas-180955007/
Subscribe to:
Posts (Atom)